The primary aim of the Euclid mission is to stringently test our current cosmological model by precisely measuring the shapes and positions of a billion faint galaxies. This ambitious goal must be achieved within a limited budget which in turn sets strict constraints on the overall mass of the satellite and the mission duration.
Designing survey telescopes has always been challenging. Photographic plates made possible for the first time quantitative measurements (position and brightness) of a large number of objects. Soon afterwards, the search started in earnest for a telescope design which would allow astronomers rapidly cover large sky areas. The Schmidt telescope design comprises a spherical mirror paired with an aspherical correcting lens. One of the most famous such telescopes, the 48-inch Palomar Schmidt telescope, covered the entire northern sky using thousands of 14-inch photographic plates and provided an invaluable discovery tool for astronomers. In the 1970s, the advent of automated plate-scanning machines meant that the first digital surveys of the sky were in fact made with photographic plates by scanning all these plates! Similar southern sky surveys were made by the UK Schmidt telescope in Australia, shown below, and these surveys were only surpassed by the arrival fully digital sky surveys like the Sloan Digital Sky Survey (SDSS). The SDSS, incidentally, bypasses the need for extremely wide-field optics by cleverly reading out the camera at exactly the rate of the earth’s rotation, but that’s another story.
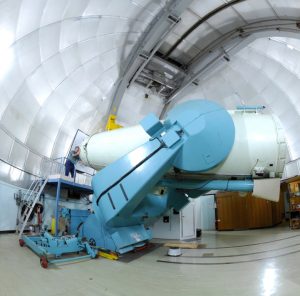
However, a significant disadvantage with Schmidt telescopes is that the focal plane — where the image is recorded — is curved, making them impractical for use with flat electronic detectors unless corrective optics are installed. In addition such telescope designs are much too large to be used in a space satellite! Remember also that one key requirement for Euclid is not simply to measure the positions, brightnesses and distances of all objects but also their shapes. For faint galaxies seen through ground-based telescopes, object shapes are dominated by atmospheric effects. On exposures longer than a few seconds, object light profiles are smeared out, severely limiting our ability to extract useful information.
For these reasons, Euclid is in space: above the atmosphere, the telescope’s shape-measuring capabilities are limited only by the satellite’s optics and detectors. This is also why telescope designs which might have been fine for an instrument lying at the bottom of the murky soup of Earth’s atmosphere are simply not good enough for space. Essentially we need a design which preserves as much as information as possible concerning the intrinsic light profile of objects. And we also need to be able to calculate how much this light profile has been distorted by presence of telescope and detector optics — usually this is done by making observations of perfect point-like sources. In astronomy terms, stellar sources fit this bill very well. But what design is this?
Automated ray-tracing revolutionized telescope design in the second half of the 20th century. Without having to cut glass, computer programs could calculate the optical performances of a telescope even before it was built. In series of papers and described at length in a classic book, the optical engineer Dietrich Korsch perfected a compact, three mirror design which had the great advantage that it features a wide field of view, few optical surfaces, and almost no aberrations over the entire field of view. It’s worth mentioning that knowing the optical performance of Euclid requires an intimate knowledge of optical properties of all surfaces. For this reason, ground testing and qualification of all components are an important part of verifying the Euclid optical design.
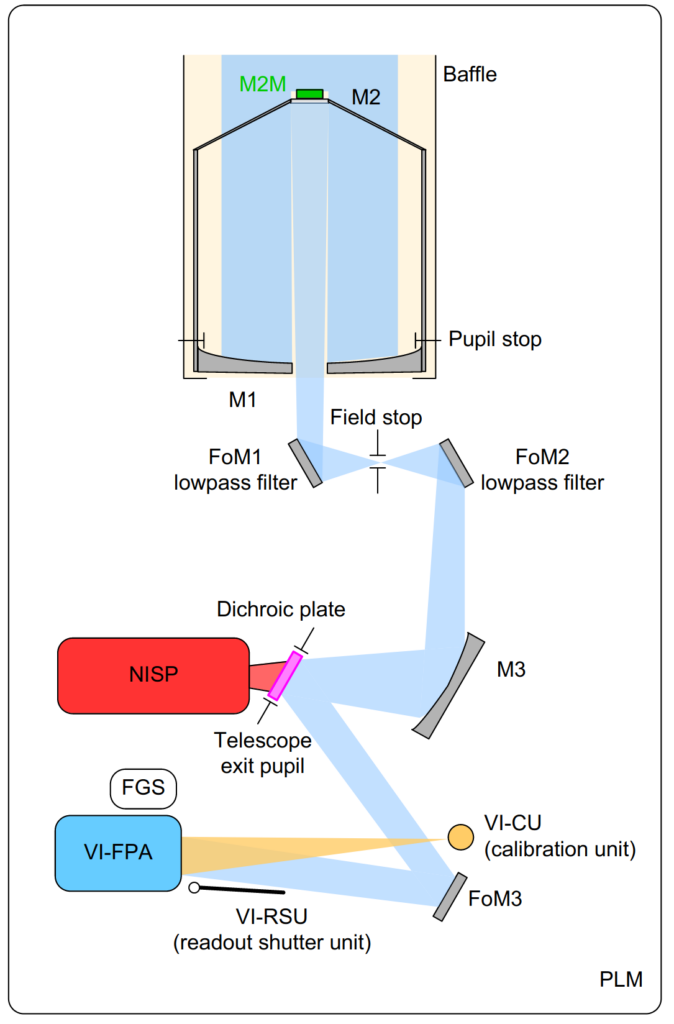
But it’s not enough to have an excellent optical design if it is not stable and image quality cannot be maintained during normal operations. So, thermal expansion and contractions must be minimised. Euclid features a Silicon Carbide (SiC) baseplate, on which all the instruments and telescope are mounted. SiC has the unique feature it expands and contracts very little with changes in ambient temperature, meaning that the path length of the whole telescope can be rigidly controlled. This very stable telescope then feeds Euclid’s main intruments (see our Blog post), NISP — a near-infrared imager and spectrograph — and VIS, a visible high-resolution camera. A special piece of glass sends redder wavelengths towards NISP and bluer optical wavelengths towards VIS, allowing both instruments to simultaneously observe the same part of the sky.
What’s the best place to put Euclid? At first, one might think, well, in orbit around the Earth. It turns out that a low-Earth orbit is a surprisingly hostile environment. In addition constant sunrises and sunsets, there are also bands of nasty charged particles, in particular in the region called the South Atlantic anomaly. Hubble Space Telescope observatory is in such an low orbit, and the unfortunate consequence is that there are ‘blackout’ periods which no observations cannot be made. In addition, there a not inconsiderable amount of background light.
There is a much better place to put a space observatory — the second Sun-Earth Lagrangian point, or L2, shown below. Here, a satellite’s location can be maintained with only a minimal expenditure of propellant. At L2, the gravitational pull of the Earth-Sun system almost perfectly balances inertial forces. Moreover, an object maintains an approximately constant distance to the Earth, making it ideal for high-bandwidth satellite communications: Euclid will need to downlink a lot of data. In this orbit will also be possible control rigorously angle the sun’s rays fall on the telescope’s sunshield: this is essential to maintain the optical stability of the telescope. These factors have made L2 one of the best locations in the solar system to place an observatory, and many future telescopes will be placed there.
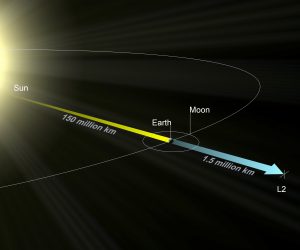
A SpaceX Falcon 9 rocket will take Euclid to L2 in July 2023. In one of the next articles we will describe in more detail how the vast flood of data from Euclid will be processed and scientific results extracted.